LIST OF ABBREVIATIONS
Secondhand Smoke (SHS)
Cardiovascular Diseases (CVD)
Chronic Obstructive Pulmonary Disease (COPD)
Sudden infant death syndrome (SIDS)
Cardiovascular (CV)
Cardiorespiratory (CR)
Over-the-counter (OTC)
Body mass index (BMI)
European Respiratory Society-American Thoracic Society (ERS-ATS)
Forced vital capacity (FVC)
Forced expiratory volume in the first second (FEV1)
Tiffeneau index (FEV1/FVC)
Mid-expiratory flow (FEF)
Heart rate (HR)
Exercise flow-volume loop (ExFVL)
Oxygen uptake (VO2)
Carbon dioxide output (VO2)
Ventilation (VE)
Respiratory quotient (RQ)
Ventilatory equivalent ratio for oxygen uptake (VE/VO2)
Carbon dioxide output (VE/VO2)
Metabolic equivalents (METS)
Anaerobic threshold (AT)
Particulate matter (PM2.5)
Maximum heart rate (HRmax)
Recovery (RC)
Oxygen pulse (VO2/HR)
INTRODUCTION
Secondhand smoke (SHS) was first reported as a danger to non-smokers by Hirayama and Trichopoulos et al. in 1981, who found increased risk for developing lung cancer among non-smoking women married to smokers1,2. Since then, scientific evidence has repeatedly associated exposure to SHS with disease and premature death in adults and children3. In adults, SHS has been associated with cardiovascular diseases (CVD), stroke, lung cancer, chronic obstructive pulmonary disease (COPD), asthma exacerbation and reproductive effects in women such as low birth weight infants4,5. Children exposed to SHS are particularly vulnerable, being put at risk for developing rhinitis, eye irritation, middle ear infection, increased respiratory symptoms such as cough, chest discomfort, wheezing, lower respiratory infections and sudden infant death syndrome6,7.
Smoking is known to adversely affect the cardiovascular (CV) system and exercise performance4 and SHS exposure is also associated with increased CV risk8–11. Studies on occupational exposure of SHS and the effect on non-smokers’ cardiorespiratory (CR) response to exercise testing are limited. Both McMurray et al. who studied a group of 8 women by smoking status, with and without SHS exposure using simulated smoke during exercise testing12 and Flouris et al. who studied SHS exposure using self-burning cigarettes to simulate bar-level exposure among healthy non-smoking adults via exercise testing7, measured short-term exposure effect of SHS on the CR system. A study by Thier de Borba et al. measured CR response through sub-maximal exertion incremental testing on a treadmill in the general population separated by smoking status13. In 2009, Arjomandi et al. studied occupational exposure to SHS in flight cabins among non-smoking flight attendants prior to implementation of commercial smoking bans and examined their flow-volume curves, diffusion capacity and lung volumes6.
Preventive policies for tobacco control include banning smoking indoors in public places and have been embraced by many countries but leave an estimated 84% of the population unprotected14. Unfortunately, even with adopted legislation, many countries have not managed to enforce the bans as is the current case in Greece15, while in others, have failed to include coverage of hospitality venues16. Individuals working in environments polluted with SHS such as hospitality venues are therefore exposed to SHS daily and chronically, highlighting the importance of examining the effect of long-term exposure on CR health.
The health effects of SHS, the fact that decreased CR response is linked to higher mortality rates in comparison to normal responses13 and the fact that many workers are still unprotected from clean indoor air legislation drive the need to further investigate the effect of long-term occupational SHS exposure on CR exercise performance.
Therefore, the current study aimed to further examine the CR response to exercise testing among healthy adult non-smokers exposed to occupational SHS and compare them to those not occupationally exposed to SHS.
METHODS
Sample population
The study recruited subjects from three Greek towns of Amfissa, Itea and Desfina in the Phocis region through open advertisement and word-of-mouth. Inclusion criteria included being age 18+ years of age, insignificant medical history, no prescribed or over-the-counter medications, non-smoker (including tobacco, nicotine products, or novel devices), and body mass index (BMI) <30. Exclusion criteria included being <18 years of age, pregnancy or lactation, presence of any chronic or acute disease or infection (<than 4 weeks prior to study) or taking any medications (<4 weeks)17. Each participant was informed of the purpose and scope of the study in detail and provided their written informed consent in accordance with Article 8, N.1599/1986 prior to beginning of the study, that was implemented following the ethical guidelines outlined by the doctorate degree program at the University of Athens Medical School.
Subjects were separated into exposed to SHS (exposed) and non-exposed to SHS (control) groups according to occupational exposure. Exposed participants were defined as those occupationally exposed to SHS for a minimum of 8 hours per day and ultimately consisted of staff that worked in cafes and bars. Those in the control group worked in public places such as schools, gyms and offices where smoking is strictly prohibited. All participants first completed a questionnaire collecting demographic, anthropometric, health and smoking history and SHS exposure information.
Equipment and Lung function tests
An ULTIMA CPX-006 system of Medical Graphics was used to measure CR parameters and gas exchange through high sensitivity gas and flow sensors in accordance with European Respiratory Society-American Thoracic Society (ERS-ATS) recommendations18 and were calibrated as per manufacturer’s protocol prior to each test. Each participant was informed of the procedure and tested at least two hours outside of work shift and at least three hours post-prandial. A physician was present during all testing.
Baseline spirometry was performed in resting conditions prior to the ergospirometry test. Variables evaluated for baseline spirometry were forced vital capacity (FVC), forced expiratory volume in the first second (FEV1), Tiffeneau index (FEV1/FVC) and mid- expiratory flow (FEF-75%)19.
Ergospirometry was performed using the Bruce 10% protocol (treadmill, incremental grade and speed; 7 stages of 3 minute durations including warm-up). Flow-volume loops and inspiratory capacity were performed at the beginning and end of all stages20. Each participant began the test by having a trial walk test on the treadmill and was closely monitored at all times. Masks were carefully fitted for each patient. A breath-by-breath respiratory gas analysis was continuously monitored and recorded along with heart rate (HR). Following the protocol, the subject was given a 3-minute recovery period and was able to interrupt the protocol at any point.
Variables evaluated were grade (%), speed (km/hr), heart rate (HR), exercise flow-volume loop (ExFVL), oxygen uptake (VO2 in mL/kg/min and mL/min), carbon dioxide output (VCO2, mL/min), VO2max, anaerobic threshold (AT), ventilation (VE, L/min), respiratory quotient (RQ), ventilatory equivalent ratio for oxygen uptake (VE/ VO2) and for carbon dioxide output (VE/ VCO2), WorkAT, WorkMAX and metabolic equivalents (METS). VO2max was defined as the point of maximum value of oxygen uptake achieved toward the end of the test. AT was estimated using V-slope method21. Maximum heart rate (HRmax) was defined as the time point at which the highest HR was reached during the test. Parameters were calculated by the system’s software using Harris-Benedict protocol22 and Wasserman equations21.
Exhaled CO, Urine cotinine and PM2.5 measurements
Non-smoking status of participants was confirmed by exhaled CO measurements taken using a Bedfont piCO Smokerlyzer23 and calibrated according to manufacturer guidelines. Values measured <7ppm were considered non-smokers24. Urine cotinine was used as the biomarker to confirm exposure to SHS in the sample population25–28. Morning urine was collected for each participant and measured using a DRG diagnostics kit29. PM2.5 measurements were conducted during winter months as bars and cafes keep doors and windows closed and exposure to SHS is maximized. The tests were made in all workplaces using a SidePAK TSI Personal Aerosol Monitor, calibrated from the manufacturer. The measurements were taken over a minimum of 10-minute intervals in each location to reflect momentary values during peak business hours (11AM-2PM and 7PM-10PM). Exposed workplaces did not have operating kitchens, burning candles or fireplaces30 therefore excluding the possibility of competing influence of PM2.5 exposure from other smoke byproducts.
Statistical Analysis
IBM SPSS statistics software version 22.0 was used for statistical analysis. Normality of distribution was determined using Shapiro-Wilks test and equality of variances with Levene’s test. Descriptive statistics of the control and exposed groups were summarized for demographic characteristics, spirometry, ergospirometry, urine cotinine concentrations and PM2.5. A correlation analysis using Spearman RHO test was used for all variables by age, height, weight and BMI. Reference time points Rest, AT, VO2max and Recovery (RC) were used for ergospirometry analysis between groups. An independent t-test determined significant differences between control and exposed groups’ with a significance level set to p-value<0.05.
RESULTS
Summary Statistics
The total sample of 60 participants consisted of two groups (control and exposed) of 30 participants. The control group was evenly distributed by gender with a majority having an education of high school or above. The exposed group had more men and a majority who had a high school or university education. The control group had similar BMI average to the exposed group (Table 1).
Exhaled CO, Urine Cotinine and PM2.5
Exhaled CO measurements of all 60 participants were <7ppm confirming their non-smoking status. Mean urine cotinine level in the control group was 518.8± 371.4ng/mL indicative of passive smoking exposure31 while the exposed had significantly higher (p=0.049) measured urine cotinine levels of 747.8± 502ng/mL than the control group. Measured workplace PM2.5 levels among the controls’ workplaces averaged from 0.01 mg/m3 to a maximum of 0.02 mg/m3. In contrast, an average PM2.5 level measured in the exposed groups’ workplaces ranged from 0.64± 0.37 mg/m3 to as much as 2.22± 1.34 mg/m3 and an independent t-test confirmed these levels were significantly higher (p-value<0.000) than the control groups’ workplaces.
Results of Lung function tests
Results of baseline spirometry parameters showed no significant differences between groups and were within normal limits. For exercise test results (Table 2) VO2 and METS against (%) predicted values showed significant differences (p<0.05) between groups at point of VO2max and RC. VCO2 also showed a significant difference between groups for AT, VO2max and RC time points. Oxygen pulse (VO2/HR, mL/beat) at VO2max in the exposed group exhibited significantly lower values against their (%) predicted than the control group by 16.6% (p=0.032) while the respective percentage difference at RC was 11.9%(p=0.011) (Figure 1).V/Q parameters VE/ VO2 and VE/ VCO2 showed relatively similar means between control and exposed groups and t-tests confirmed there was no difference (p-values>0.05), as did the WorkAT and WorkMAX t-test results.
Figure 1
Mean values (±SE) of maximum oxygen uptake over heart rate (VO2/HR) expressed as (%) predicted values in Control and Exposed at points of anaerobic threshold (AT), maximum oxygen uptake (VO2max) and recovery (RC)
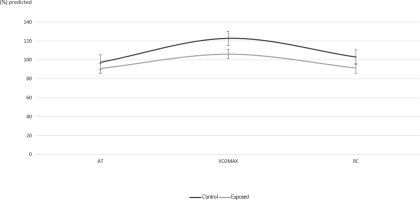
Table 2
Comparison of ergospirometry between Control and Exposed
DISCUSSION
Our results indicated that ergospirometric parameters are affected by chronic occupational SHS exposure. We found that chronic exposure to SHS has a negative impact on VO2, VCO2, METS and VO2/HR at points of VO2max and RC. These findings indicated that non-smoking, otherwise healthy, workers exposed to SHS in the workplace show lower exercise performance from an average of 6.9% to 14% than workers not exposed to SHS. In addition, non-smoking status was confirmed by exhaled CO measurements, exposure to SHS confirmed by urine cotinine and workplace environmental pollution through PM2.5 concentrations.
Non-enforcement of smoking ban legislation in Greece leads to SHS exposure outside work that could explain urine cotinine levels found in the control group especially since their measured CO indicated they were in fact, non-smokers24. Although this could also be the case in the exposed group, the fact that they exhibited significantly higher levels of urine cotinine (p<0.05) and environmental PM2.5 (p<0.000) as well as significantly decreased exercise performance (p<0.05) could be attributed to supplemental SHS exposure in their workplace.
Oxygen pulse is a reliable index for assessing heart function21,32. It depends on the volume of oxygen extracted by the peripheral tissues and is the volume of oxygen uptake by the pulmonary blood during the period of a heartbeat21. It is the product of stroke volume and arterial-mixed venous O2 difference21. Therefore, a decrease in stroke volume, anemia and carboxyhemoglobinemia are factors that lead to a decrease in oxygen pulse21. Since the current study population had no history of heart disease or anemia, the lower values recorded in the exposed group could be explained by the formation of carboxyhemoglobinemia through inhalation of CO present in the SHS mixture as it is the case with cigarette smoking. Carboxyhemoglobinemia causes a leftward shift in the oxyhemoglobin dissociation curve, making it more difficult for oxygen to dissociate from hemoglobin (Hb) at any given partial pressure of oxygen (PO2). Capillary PO2 then falls rapidly to its critical value and lactic acidosis occurs at a reduced level of Work reducing peak VO2 and AT and consequently adversely affecting exercise tolerance21.
Earlier studies that examined SHS exposure and exercise performance are in agreement with this study’s findings although McMurray et al. and Flouris et al. studied the short-term exposure effect of SHS while Their de Borba studied the effect in the general population whereas the current study measured the long-term occupational exposure to SHS. McMurray et al. examined short-term exposure to SHS and found that involuntary inhalation of SHS lowers maximal exercise capacity and submaximal response among smokers and non-smokers12 while Flouris et al. reported that a single hour of exposure to SHS adversely effects VO2max in healthy non-smokers33. Thier de Borba et al. also found that long-term SHS exposure affects VO2max in the general population as it was significantly lower in passive non-smokers than in non-exposed non-smokers and very interestingly found no significant differences in VO2max between smokers and passively exposed non-smokers13.
In regards to spirometry, normal flow-volume loops were found with no significant differences between groups. In contrast to these findings, Arjmandi et al. who examined healthy flight attendants found significant decreases in spirometric parameters (mid- and end- expiratory flow), diffusion capacity and lung volumes6. Their findings can be attributed to the significantly different environment represented by the flying aircraft cabin, where changes in altitude, pressure fluctuations as well as exposure to ozone, CO2 and various other pollutants (fungi, bacteria, protozoa) exert physical strain on a traveler’s body34. While partial pressure of oxygen in the atmosphere remains at 21%, the atmospheric pressure in the air cabin drops at high altitudes and results in lower partial pressure of oxygen35 and the consequent hypobaric hypoxia could be the underlying mechanism for decreased diffusion found in their study. Air trapping could also be explained by Boyle’s law; as pressure falls in the air cabin while temperature remains constant the volume of gas in the bodies’ cavities increases36.
Although the control group was not exposed to SHS in the workplace (PM2.5 measurements < 0.024 mg/m3)31 and exhaled CO indicated non-smoker levels24, urine cotinine levels can only be explained by SHS exposure in daily activities other than at work. Wendy Max et al., found that despite many years of tough tobacco-control policies in California, people continue to be exposed to SHS at home and in the workplace16.
PM2.5 has been established as a hazard in the environment linked to CVD and increased mortality risk37. According to Haberzzetl et al., PM2.5 induces CVD through decreasing antioxidant capacity of the lung38. Therefore, enhancing the antioxidant defense in the lungs could reduce risk of CV injury associated with PM2.5 exposure38. Cigarettes emit high levels of PM2.5 when smoked39. Results of the current study showed the exposed group were at times exposed to substantial amounts of PM2.5 in the workplace and also confirmed failure of smoking ban enforcement in bars and cafes in Greece, while control workplaces had very low PM2.5 readings. These findings are supported by Wortley et al., who found that SHS exposure varies with occupation; with blue collar and service workers exhibiting higher and white collar workers lower levels of exposure40. High SHS exposure has also been found among casino workers8.
SHS increases the risk of lung cancer and coronary heart disease by up to 30%41. Repeated studies at cellular level and among animals have shown that exposure even at the lowest levels of SHS increase the risk on the CV system11. Other studies have shown that exposure to SHS before 25 years of age have higher risk of developing lung cancer than those exposed after 2541. Smoking bans have been proven to be associated with reduced coronary episodes11. Raitakari et al. found that endothelial function, a marker of arterial health, was significantly better in young adults who had withdrawn from regular exposure to environmental tobacco smoke than in passive smokers42. However, the evidence of respiratory health impact of indoor smoking bans is not as robust9 and findings of the current study support the enforcement of bans to improve respiratory performance of non-smokers which could have a substantial impact on prevalence of disease, quality of life and consequently an economic gain37.
When particular workplaces such as hospitality venues are exempt from implementing the smoking ban legislation, it provokes discrimination against workers; offering health protection to those who work in smoke-free workspaces while those exempt from the law are left unprotected and at risk for serious chronic diseases while their basic human43 and labor rights are violated44–46. Article 7 of the International Covenant on Economic, Social and Cultural rights44 and the Lisbon treaty45 state that all working individuals must be protected from harm and enjoy safe and healthy work environments while Article 8 of the WHO Framework Convention for Tobacco control specifically outlines protection from SHS46.
It is acknowledged that repeated studies are needed to assume any generalizability due to the current study’s limited sample size. Uneven distribution of gender in the exposed group was also a limitation, seemingly caused by more men being employed in the hospitality industry in Greece than women. PM2.5 levels in workplaces were limited to reflect momentary exposure in the workplace and hence may not be directly associated with recent exposure to SHS.
CONCLUSION
Among healthy adult non-smokers, chronic SHS exposure of those who worked in hospitality venues contributed to lower exercise performance. Its impact on chronic disease development should be further explored. These results add to the evidence of the importance of enforcing clean indoor air legislations and protecting nonsmokers from exposure to SHS.